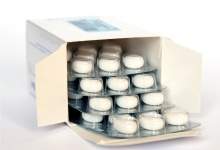
Earlier this year, a research team at Michigan State University (MSU) managed to reveal, for the first time, the crystal structure of TSPO, a protein associated with several forms of anxiety disorders which is also used to aid in imaging areas of inflammation in the brain, where it is found at high levels. By identifying the structure at the atomic level, the researchers hope to be able to pinpoint where drugs or ligands may interact with the protein, which could – eventually – lead to new, better anti-anxiety medicines with fewer side effects, as well as improved diagnostics for brain inflammation.
But it’s not going to be an easy or a quick road, according to the researchers behind the breakthrough, as solving TSPO’s structure, a process which has taken six years, is only the first step in their quest to understand how the protein actually works.
One of the key breakthroughs the team made was crystallising a mimic of a human TSPO mutation, which is common in people with conditions such as bipolar disease. The mutant protein was found to bind cholesterol less strongly, perhaps because the structure they determined is altered and more ridged, limiting cholesterol interaction. This is significant because TSPO may play a role in cholesterol entry into mitochondria, where it is converted into hormones that are essential for our bodily functions. If this process isn’t working properly, these hormones can’t be made.
TSPO’s structure: six years in the discovering
So how did Shelagh Ferguson-Miller, university distinguished professor of biochemistry and molecular biology at MSU, and her team, made up of postdoctoral researchers Fei Li and Jian Liu and professor Michael Garavito, get to this point, which no researchers before them had managed to reach? "With difficulty," Ferguson-Miller laughs, adding that the entire process of solving the protein’s crystal structure has taken around six years.
In a nutshell, after crystallising the protein, the team used X-ray technology to create an image of TSPO at a molecular level, which gave them an increased understanding of how the protein interacts with cholesterol and how this relationship affects the creation of essential hormones for the body – but the whole process was much more complicated than it might sound.
How well do you really know your competitors?
Access the most comprehensive Company Profiles on the market, powered by GlobalData. Save hours of research. Gain competitive edge.
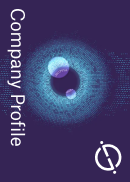
Thank you!
Your download email will arrive shortly
Not ready to buy yet? Download a free sample
We are confident about the unique quality of our Company Profiles. However, we want you to make the most beneficial decision for your business, so we offer a free sample that you can download by submitting the below form
By GlobalDataSee Also:
Scientists from Vanderbilt University have opened new frontiers in obesity research by bioengineering bacteria.
"Membrane associated proteins are very hard to purify and very hard to handle, so the initial challenge was to get them to crystallise in the first place," explains Ferguson-Miller. "Then we had to use a variety of approaches to collecting and analysing the data and solving the structure. At every level it was challenging, requiring cutting edge technology and including many failures."
Fortunately, each member of the team involved brought different areas of expertise to the table. "Dr Garavito is a long-term crystallographer, so he was extremely helpful in analysing difficult data, while Fei Li and Jian Liu figured out how to produce, purify, crystallise and collect data on the protein with the help of robotic techniques," Ferguson-Miller explains. "I was the day-to-day consultant who tried to keep everyone encouraged to continue."
Next steps
The point the team has reached now – knowing the protein’s crystal structure – though, really is just the beginning. "The problem is that TSPO is a protein whose exact function is still not known," Garavito explains. "And without knowing its function, it’s hard to know how to use it as a drug target."
This is something the team will continue to work on at various levels, while at the same time attempting to identify the structure of proteins from human cells, rather than from bacteria. (The latter is what they have used in their work up until now because bacterial versions of the protein are closely related to their human counterparts – and easier to work with).
"One of the things we want to do is get a really good structure of the human protein so that it can serve as a more accurate basis for drug design," Ferguson-Miller stresses, admitting that, just like their previous work, it’s unlikely to be easy. "The human protein is hard to deal with for a variety of reasons, which is why lots of researchers try to crystallise bacterial versions instead of human versions, but we will try hard to get the human and the mouse version, as the latter is a great experimental system, too."
That said, however, understanding the bacterial protein’s structure is a great first step. "Having the protein’s structure allows computer aided drug design to work better, which then potentially speeds up the process of finding compounds that interact very specifically and very strongly with this protein," Ferguson-Miller explains. "There are wonderful computational methods for docking proteins with other proteins and drugs with proteins, so those techniques can be used to try to understand how this protein interacts and what it’s doing."
One area of immediate application – before the research can start to be used in the anti-anxiety field – is in positron emission tomography, or PET imaging, where compounds that bind to TSPO are used to detect areas of inflammation and injury in the brain, due to the fact that TSPO is highly expressed in those regions. "Even better, more specific ligands will be helpful in this method, and potentially for treating neuro-inflammatory disease as well," says Ferguson-Miller.
Wider applications
The team’s research could also have wider applications in the future. "The methodology we used to get this protein’s structure isn’t new but our success with that methodology will probably inspire other people to use it and to get structures of other membrane proteins," Ferguson-Miller says.
Biosimilars could save patients and health systems billions.
"Many membrane proteins are of great interest to the pharmaceutical industry and yet people don’t try to crystallise them because they know how hard it’s going to be. It’s also hard to get continuing funding for projects that haven’t got an assured successful outcome. Hopefully, our work can in fact illustrate that some of these more difficult proteins really can be attacked and that, in the future, could potentially help researchers understand more proteins."
In the meantime, the anti-anxiety drug sector can start to get a little bit excited. "Leading to better drugs is always a game of chance and luck, but I think our work may at least give us a new understanding of one factor that’s a contributor to anxiety," Ferguson-Miller concludes.
"We’re hoping this new understanding, and further insight into how TSPO functions, will help direct the industry to possibilities in developing new drugs – but it’s still a long way away."