
It takes a lot of poppies to make serious painkillers. Morphine is classed as an essential medicine by the World Health Organization, but it wouldn’t exist without Papaver somniferum (better known as the opium poppy). Around 100,000 hectares of the flowers are cultivated every year – primarily in Australia, Spain, France, India, Turkey and Hungary – to meet demand for opioid medication worldwide. Approximately 800 tons of the natural opiates morphine and thebaine are extracted from the harvested straw, some of which are then chemically converted into higher value drugs, such as codeine, oxycodone and hydrocodone.
Although fields of beautiful blooms sound idyllic, industrial poppy farming isn’t easy.
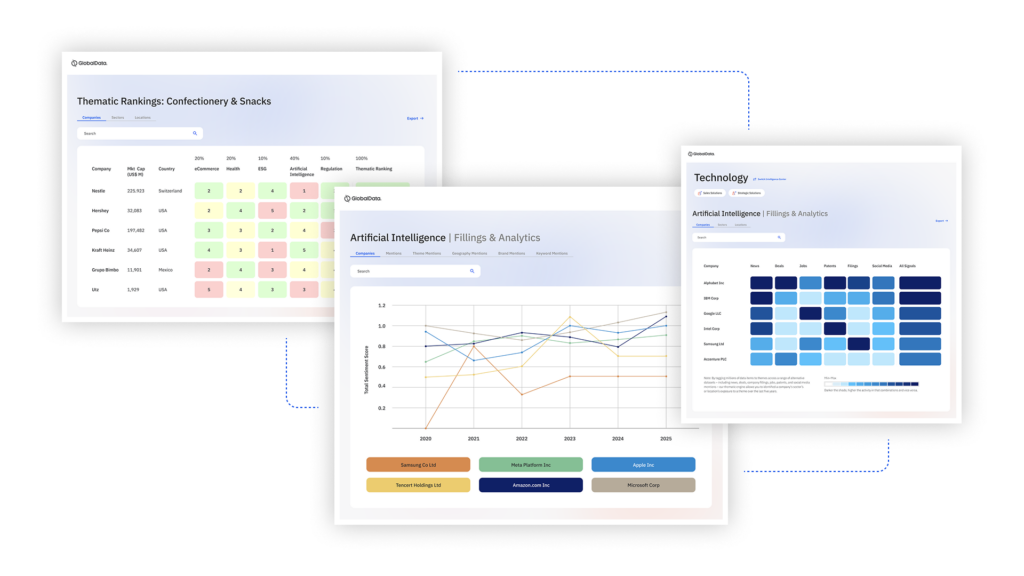
US Tariffs are shifting - will you react or anticipate?
Don’t let policy changes catch you off guard. Stay proactive with real-time data and expert analysis.
By GlobalDataPests, disease and poor weather conditions can dramatically impact the yield. Plus, growing drugs from plants takes a long time and the poppies themselves contain very low levels of the active ingredient. If there is a spike in demand for opioid medicines, the supply chain can struggle to respond fast enough. These issues mean problems for the pharmaceutical industry, which in-turn means drug shortages for doctors and patients.
Medicines derived from nature (as around 40% of our drugs are) are particularly vulnerable in times of uncertainty. During the Covid-19 crisis, we’ve seen that supply chain problems can have a human cost. In the spring, the US Food and Drug Administration reported a shortage of the opioids needed to safely keep patients on ventilators.
There could be a better way of making the rare compounds found in nature and avoiding similar supply chain issues, says Stanford University adjunct professor Christina Smolke. In 2015, she and a small team of researchers proved that opioids could be produced using genetically-modified yeast.
Reimagine fermentation
Smolke’s breakthrough involved a process we’ve known about for centuries: fermentation. Give brewer’s yeast a little sugar and under the right conditions it’ll reward you with alcohol and carbon dioxide.
Smolke figured fermentation had the power to speed up drug manufacturing – with yields seen in day or weeks, rather than months or years as with crop farming. It would mean supply could be easily increased if there was an unexpected event.
But plant-derived medicines are made from more complex stuff than ethanol or carbon dioxide. “If you look at the types of molecules plants make, they’re incredibly complicated from a structural perspective,” says Smolke. “The way they are able to survive and interact with their environment is through chemistry.”
These molecules are almost impossible to replicate in the laboratory using synthetic chemistry so Smolke, who has a chemical engineering background, instead turned to synthetic biology. First, she identified which plant enzymes are responsible for the chemical reactions that lead to the useful compounds.
Then it’s a case of making the genetic recipe for these chemicals and translating it into a language the yeast can understand. Synthetic DNA is then inserted into the yeast, which tells it to make the desired compounds out of the sugar and amino acids the scientists feed to it.
To coax the yeast to make the opioid hydrocodone, the team translated 23 proteins from the poppy into the microbe. After her initial breakthrough, Smolke formed start-up synthetic biology company Antheia to focus on creasing ever-more complex medicines using yeast.
It’s not the first time a microbe has been used to make medicine. In 1982, Eli Lily introduced synthetic insulin Humulin to the market, a breakthrough drug for people with diabetes. Before, insulin had to be extracted from the pancreases of cows or pigs. This caused allergic reactions in some patients as animal insulin isn’t an exact match for the hormone produced in healthy human pancreases.
Humulin is made by introducing human DNA into a bacterial host cell. As the bacteria multiply, the medicine is produced. Human insulin can now also be made using yeast cells and fermentation. And since 2014, Sanofi has also used yeast to produce artemisinic acid to make the anti-malarial artemisinin, which is usually sourced from the sweet wormwood plant.
Borrow from nightshade
In August 2020, Smolke and colleagues announced in Nature that they had successfully produced neuromuscular blockers used in Parkinson’s disease and intestinal disorders. These chemicals were the tropane alkaloids hyoscyamine and scopolamine, which are naturally found in the nightshade family of plants.
As with the poppy, cultivating nightshades is subject to global supply risks, such as environmental disasters and the ongoing pandemic. Shortages of alkaloid-based medicines are unfortunately common.
To achieve tropane alkaloid synthesis in yeast, Smolke and her team had to overcome several challenges. As before, they first needed to work out which enzymes the nightshade plant uses to make the compounds and how to get those to work in a yeast host.
But the biggest hurdle was realising the critical chemical reactions required to make tropane alkaloids are segregated across different subcellular compartments, cells or tissues in the plant. It would be tough to recapitulate this in single-cell yeast.
“In many cases, it’s not enough just to get the protein made because it won’t have the correct kind of biochemical environment for it to actually perform its reaction,” says Smolke. “A lot of times where these types of projects fail you can identify the enzyme, but you can’t get the yeast to make it in a way that the reaction actually happens.”
After a gruelling coding process involving 26 genes from ten different organisms, they were able to create six different subcellular compartments within the yeast cell to make sure the right chemical reaction would occur at the right time.
“What we also did beyond distributing the enzymes was incorporating other proteins like transporters that could be localised to the membranes of these compartments,” Smolke explains.
This allowed for more efficient routing of the metabolites from one set of chemistries to the next. In doing so, the team transformed the yeast into a chemical factory, in which different synthesis steps are conducted in separate reactors to ensure each reaction has the optimum conditions.
The Nature paper is further proof that brewer’s yeast can be a platform for making some of nature’s most valuable and complex molecules. The next step for Smolke will be convincing the pharmaceutical industry that this could be the future of drug manufacturing. She and her team will need to prove the technology can mass-produce molecules faster, cheaper and more reliably than the original farming methods.
In the latest work, only a few milligrams of tropane alkaloids per litre of yeast culture were produced – not yet a competitive alternative to production through plant extraction. However, Smolke says Antheia is constantly tinkering with the yeast to improve its efficiency so a larger amount of compound over a given period time is produced.
“As these examples scale, we’ll see there are new ways of structuring supply chains that will bring more resiliency and control,” Smolke says. “This is going to open up the pharmaceutical industry.”