
The potential for breakthrough cell and gene therapies to transform healthcare cannot be underestimated. Some of the most complex and sophisticated medical treatments in development involve reprogramming a patient’s immune system to fight and even prevent the development of diseases, which are either difficult to treat or untreatable using traditional medicine.
Gene therapy aims to treat diseases by replacing, inactivating or introducing genes into cells— either inside the body (in vivo) or outside of the body (ex vivo). An example of the in vivo approach is Novartis’ Zolgensma® (onasemnogene abeparvovec), a recombinant adeno-associated virus (AAV9)-based gene therapy designed to deliver a copy of the gene encoding the human SMN protein.
Cell therapy aims to treat diseases by restoring or altering certain sets of cells or by using the cells themselves as a therapy. Some examples of cell therapy products include stem cells and stem cell-derived products, such as those from hematopoietic, mesenchymal, embryonic, and umbilical cord blood, cancer vaccines and immunotherapies. Cells that are altered genetically are tagged under Gene-Modified Cell Therapy in accordance with the standards by the Alliance for Regenerative Medicine (ARM).
Extraordinary potential
It’s still very early days for these therapeutic modalities and there are only a few approved treatments in this space right now, but results in many of the studies that have been conducted over the past year or two have been powerfully positive and show the extraordinary potential of these therapeutics, collectively known as advanced therapy medicinal products (ATMPs).
According to market intelligence and research firm GlobalData, five ATMPs gained FDA approval in 2022 – two cell therapies and three gene-modified cell therapies – a record high. Janssen’s Carvykti® (ciltacabtagene autoleucel), a gene-modified cell therapy as a treatment for refractory multiple myeloma, is projected by GlobalData analysts to become a blockbuster drug and the most commercially successful of the ATMPs approved in 2022. Altogether, there are 10 gene-modified cell therapies and seven gene therapies approved and marketed in some or all of the major geographies, including the EU, Japan, US, Israel and the UK.
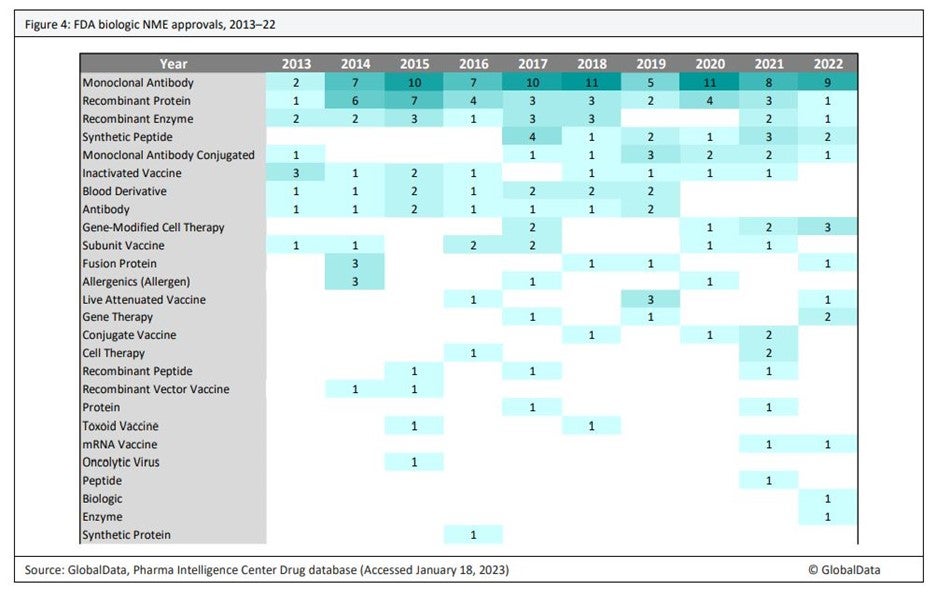
“We predict that this number will soar in the near future. We anticipate that more than 100 more gene therapies and gene-modified cell therapies will be approved over approximately the next six years,” says Fiona Barry, editor-in-chief and associate director at PharmSource, a GlobalData company covering contract manufacturing in the pharmaceuticals industry. “Clinical trials for gene therapies and gene-modified cell therapies show steady growth over time, reflecting growing scientific knowledge and industry confidence in gene therapy and the first wave of regulatory approvals.”
One powerful gene-modified cell therapy that has demonstrated significant impact in recent years, particularly in treating certain cancers, is a therapy that works on a part of the immune system called T cells. This therapy works by removing a type of immune system cell – T cells – from the patient’s blood, genetically modifying it in a lab to make them effective at targeting and killing antigen-expressing cancer cells and then infusing them back into the patient.
First cell therapy products for solid tumours in 2023-2024
Sakis Paliouras, associate director, oncology and hematology at GlobalData Healthcare says CAR-T has transformed the treatment of hematologic malignancies such as B-cell non-Hodgkin’s lymphoma, acute lymphocytic leukemia, and multiple myeloma. “Novartis’ Kymriah® and Gilead’s Yescarta® were the first products to be approved and have resulted in highly lucrative sales for the companies,” he says.
Success of CAR-T, albeit in the relapsed setting, has turned the spotlight on this technology and how it can be brought forward from third-or-fourth line treatment to second or even first line treatment to save a patient going through several rounds of debilitating chemotherapy or radiation therapy beforehand. According to GlobalData, about three quarters of Phase I–III gene therapy and gene-modified cell therapy trials are for oncology, but the gene-editing technologies behind CAR-T, including CRISPR, are also being trialled to see how effective their impact is on other disease indications, including for genetic disorders like Sickle Cell and Cystic Fibrosis, as well as hematological and central nervous system conditions. As for solid tumours, Paliouras says cell and gene therapies (CGTs) are soon expected to make an impact.
He says: “The first gene therapy products for solid tumors were approved more than 10 years ago, which in the US was Amgen’s Imlygic® for metastatic melanoma, but the clinical impact has been limited. Market authorization for cell therapies in solid tumors is eagerly anticipated. GlobalData anticipates Iovance Biotherapeutics’ tumor-infiltrating lymphocyte (TIL) therapy Lifileucel and Adaptimmune’s TCR-T therapy Afami-cel to be the first cell therapy products to be approved for solid tumors in 2023-2024.
“Such approvals could spark a new wave of R&D investment into the TIL and TCR technologies that could expand the field of CGT further.
Genetic material delivery method in the spotlight
By the end of 2023, the cell and gene and gene-modified cell therapies are expected to be worth $7 billion, GlobalData forecasts suggest this represents an increase from $4.3 billion in 2022. By 2029, GlobalData projects these revolutionary treatments could be generating $67 billion for the industry. The potential of these new therapeutics – and the financial rewards attached – puts the spotlight on the delivery technology critical for introducing nucleic acid payloads into target cells or tissues.
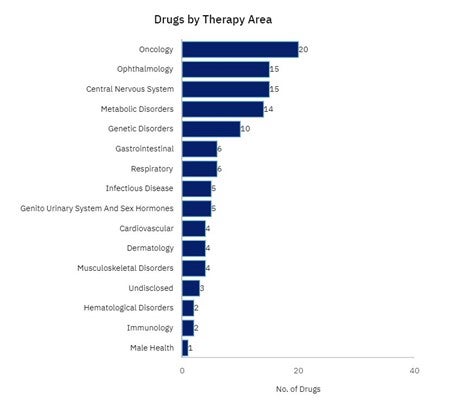
Currently, all approved ATMP therapies use viral vectors as the delivery platform. These are based on modified retroviruses, lentiviruses, adenoviruses (Ads) or adeno-associated viruses (AAVs). Here, a patient’s T cells are isolated via leukapheresis and genetically engineered ex vivowith viral vectors, encoding the CAR transgenes designed to recognise tumour-specific antigens. Viral vectors are a popular mode of gene delivery because of their high transduction levels and stable expression, but Angela Zhang PhD, group leader, cell and gene therapy product management at Precision NanoSystems, says they have some limitations.
“Viral vectors have gone through the regulatory rigour and are deemed safe,” says Zhang, who is one of the leaders in lipid nanoparticle-based delivery product strategies in cell and gene therapies at the Vancouver-based biotechnology company. “The viral vector field has advanced tremendously, but there is a strong desire to improve safety further and continue to investigate the viability of alternatives.”
There are other drawbacks to using viral vectors. Their cargo capacity makes it difficult to implement for larger new-generation nucleases (such as prime editors). Furthermore, production can take over a month compared to hours for LNPs, which pushes up costs. Most significantly for the industry, there is currently a supply chain crunch.
“A shortage of viral vectors exists due to insufficient manufacturing capacity, an inefficient manufacturing process, and the requirement for complex specialist facilities. This shortage will be worsened by a coming wave of gene therapies and gene-modified cell therapies, and by the enormous number of doses to be manufactured of certain COVID-19 vaccines that require a viral vector,” suggests a GlobalData report ‘The Outlook for Viral Vector Contract Manufacturing: Gene Therapies, Cell Therapies and Covid-19 vaccines’ published in May 2021.
The report said that at the time of publishing viral vector capacity was less than 1% of what it needed to be to fulfil market demand for manufacturing gene therapies, gene-modified cell therapies, and recombinant vector vaccines.
In addition, there is substantial interest in the engineering of cells for transient expression of a therapeutic protein, and for gene editing to remove proteins of interest, and these applications are less suited for viral vectors.
The rise of non-viral alternatives has given rise to the desire to look at ways of making certain types of cell therapies more transient – hitting that sweet spot between extremely stable and very transient expression is an area that is actively being investigated using LNPs.”
The convergence of these factors has seen interest ramp up in emerging non-viral delivery technologies, including electroporation and lipid nanoparticles.
Lipid nanoparticles: a promising non-viral vector alternative
Physical and chemical non-viral gene delivery systems for ex vivo genetic modification offer advantages over viral vectors, including a lower risk of immunogenicity.
Electroporation is a widely used physical cell transfection method to move genetic payloads across the cell membrane where an applied electrical pulse temporarily disrupts the phospholipid bilayer of the cell membrane, resulting in the formation of pores that allow a diverse range of cargo molecules to pass into the cell. Once optimal electroporation conditions are established, it can be easy to execute with versatility in payload size and utility across all cell types. But a major drawback of electroporation is the cellular cytotoxicity caused by the high-voltage pulses that can induce changes to the lipid membranes, proteins, and DNA, causing oxidative damage through the generation of reactive oxygen species.
As Zhang says: “Cells don’t like being zapped.”
This has direct implications on the cell viability which is further exacerbated by more complex sequential genetic manipulation and strategies to deploy repeated dosing.
Studies have shown that using lipid nanoparticles (LNPs) don’t lead to the same dramatic trade-off as for electroporation between efficiency and cell viability.[i] Clinically validated for gene therapies, particularly RNAi drugs, LNPs protect mRNA against degradation, and the successes of the Moderna and BioNTech SARS-CoV-2 mRNA vaccines have demonstrated that they are an effective drug delivery system. LNPs were also used in the first FDA-approved RNAi therapeutic, ONPATTRO® in 2018, the first small interfering RNA-based drug to treat patients with polyneuropathy caused by a rare, debilitating and often fatal genetic disease characterised by the buildup of abnormal amyloid protein in peripheral nerves, the heart, and other organs.
More recently, the gene-editing biotech company Intellia Therapeutics, which focuses on CRISPR-based lifesaving therapies, has demonstrated the in vivoclinical efficacy and safety of LNPs. To date, the pioneering biotechnology company has used its LNP technology to investigate a CRISPR ex vivo treatment candidate – NTLA-5001 – for patients suffering from Acute Myeloid Leukemia. This autologous cell therapy candidate is designed for AML patients with the HLA-A*02:01 allele and whose tumors carry the Wilms’ Tumor 1 (WT1) antigen, which is widely overexpressed in AML and other cancers.
Zhang says LNPs are gentle on the cells, helping to avoid the challenges created by other technologies. They are also transient and can potentially improve safety for patients. Although this cannot be guaranteed as there is no cell therapy currently on the market using LNPs as the delivery platform.
Zhang adds: “Production platforms suited for clinical and commercial scale manufacturing of LNPs
overcome potential scalability limitations of electroporation and are thought to be less costly. But optimising lipid nanoparticle formulations to support CAR-T and other novel immunotherapies requires an in-depth understanding of the analytics and parameters of an LNP’s unique components as well as having the right manufacturing systemsto go from discovery to the clinic.”
Viral vectors won’t be replaced anytime soon. There are currently almost 6,100 cell, gene-modified and gene therapies in development, from pre-registration to phase III and of these just 327 are being manufactured using a non-viral approach. But this is a fast-evolving space, says Zhang. “Increasingly, we are seeing companies utilise the combination of LNPs and viral vectors to create gene and cell therapies. I am excited to be part of a company leading the way to the future of lifechanging medicine.”
[i] Cell death due to electroporation – a review, National Library of Medicine