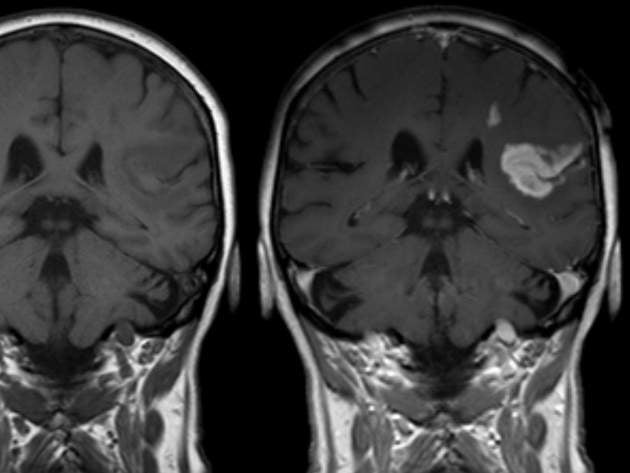
Nanoparticles have long been considered to have important implications for medicine. Unimaginably small in scale (you could fit between 1,000 and 100,000 across the width of a human hair) these ultrafine, lab-engineered particles are now in demand across multiple applications.
They can be used, for instance, for targeted drug delivery, in which they are loaded with drugs and unloaded at the requisite part of the body. This is particularly useful when treating tumours, as it reduces the side effects associated with traditional chemotherapy.
They can also function as targeted contrast agents in magnetic resonance imaging (MRI), improving specificity. And while work is still in its early translational stages, some scientists are looking to combine these two uses (therapeutic and diagnostic) into a third class of application: ‘theranostic’.
Research limitations
Since the first nanodrug, Doxil, was approved in 1995, there has been an explosion of research in the field. To date, the US Food and Drug Administration (FDA) has approved over 50 imaging or therapeutic nanoparticles, with the number of clinical trials underway tripling between 2013 and 2016.
It is arguable, however, that the true benefits of nanoparticles in drug development have yet to materialise. For one thing, it can take a decade or more for preclinical research to hit the market. For another, there are still many unknowns surrounding nanoparticles’ behaviour, along with problems around toxicity. If their potential is to be realised, it will be critical to overcome some of the safety challenges.
How well do you really know your competitors?
Access the most comprehensive Company Profiles on the market, powered by GlobalData. Save hours of research. Gain competitive edge.
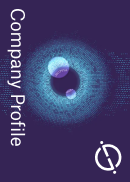
Thank you!
Your download email will arrive shortly
Not ready to buy yet? Download a free sample
We are confident about the unique quality of our Company Profiles. However, we want you to make the most beneficial decision for your business, so we offer a free sample that you can download by submitting the below form
By GlobalDataThe central issue is that not all nanoparticles are made alike: some are more biocompatible than others, and they can interact with the immune system in unpredictable ways. This means researchers can spend months or even years developing a particle, without knowing exactly what effect it will have on the human body.
“The smaller the particle, the higher the ratio between the surface and area, and this high surface ratio leads to a high reactivity with cells,” says Inès Mottas, a biomedical researcher at the University of Geneva. “Shape also plays a role, in that the round shape is less likely to lead to membrane disruption. Another point is the charge on the particle surface – because the cell membrane is negatively charged, there will be a high interaction with positively charged particles. You have to find a balance between what you want and what you can do, because everything plays a role.”
Three tests in one
Mottas, together with colleagues at the Universities of Geneva (UNIGE) and Fribourg (UNIFR), recently developed a screening technique that could make researchers’ lives much easier. This technique can select the safest nanoparticles in a matter of days, as opposed to months.
It tests nanoparticles against three parameters: their toxicity (do they kill healthy cells?); their interaction with macrophages (are they bypassed or ingested?); and their overall effect on the immune system (do they trigger an inflammatory response that would lead to side effects?) While previously all these parameters were tested separately, the beauty of Mottas’ technique is that it addresses the three in parallel.
“In the past, we had to do this step by step, which is time-consuming and requires a lot of different materials, since you need to repeat your results with different batches,” says Mottas. “I set up this protocol to ensure we have data as soon as possible in only one step.”
The technique, based on flow cytometry, analyses macrophage cells one by one with lasers to see whether they are alive (a measure of cytotoxicity). It also includes staining to assess whether they have been activated (associated with inflammation). Finally, it measures the uptake of nanoparticles.
“For every single cell you get the data, so you can know whether it’s alive and if it took up particles,” says Mottas. “Sometimes it looks perfectly good but it didn’t take particles, and sometimes it took up particles but they’re toxic. So it’s important you can really correlate the number of particles they took up with whether they’re dead or alive or activated or not.”
Reproducing the results
Because this test is highly reproducible, it allows researchers to easily perform quality control checks – for instance seeing whether there is any variability between batches – as well as ruling out false positives and false negatives.
“When you begin to test a nanoparticle, you don’t know what factors plays a role,” says Mottas. “So first we test the particles, and then when we receive a second batch we have to make sure it acts exactly the same way as the one before. There might be discrepancies due to temperature, or to contaminants such as leftover solvents, which are not biocompatible.”
Material choice is also critical. Mottas points out that, wherever possible, researchers ought to opt for biodegradable particles that do not stay in the body.
“Gold silica and iron oxide particles, for instance, are not destroyed by the body and we do not know exactly what will happen,” she says. “So from my point of view, the best thing to do is to perform the proof of concept with different materials, but then to go for particles we know can be easily digested.”
Future applications
Their technique, recounted in the journal Nanoscale in February, is part of the work carried out within the National Centres of Competence in Research (NCCRs) ‘Bio-Inspired Materials’ programme. Clearly, it will not only save researchers time – it will save them money. By shaving weeks off the time required for screening, it could open up possibilities that were once seen as impracticable.
Nowhere is this more the case than in the field of personalised medicine. Mottas’ team believes that, by isolating tumour cells from a patient, it should in theory be able to assess which particles suit them best.
“Our test is now performed on a macrophage cell line, but we can imagine doing it with every type of cell, even those derived from humans,” says Mottas. “So if we are looking to adapt the treatment on an individual basis, we’d take cells from each patient and test the different nanoparticles against them. This means we’d be able to determine for each specific patient which particle is the best.”
While this particular application is hypothetical, the technique is already being used with great success within research. Since it is suitable for biology and chemistry labs alike, it might help bridge some of the conceptual divides between the two fields.
Mottas says her team uses the protocol every time they receive new materials from collaborators. To date, projects have included new strategies for improving cancer vaccinations, along with cancer treatments that specifically target the lymph node.
“The first time I reviewed the research, I told myself it’s impossible to predict if the nanoparticles will interact well with the immune system or not,” says Mottas. “But it’s easy to test with this approach, and in 24 hours I have my answer.”